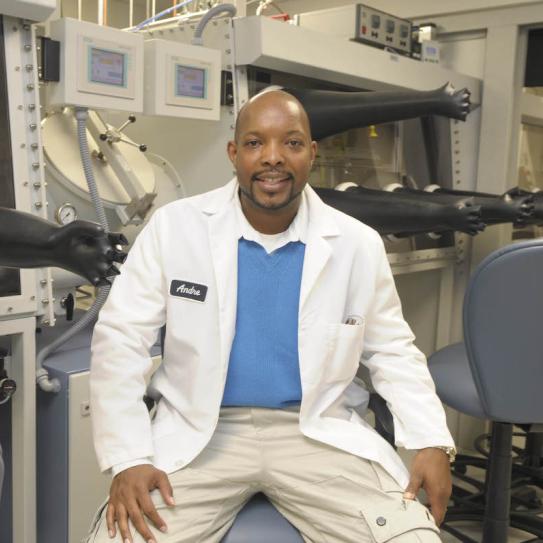
Watch: André Taylor discusses his research on Perovskite Solar Cells with Hari Sreenivasan on scitech now
Journal Articles
F. Antonio, M. Beromi, T. Goh, N. Hazari, J. Kong, M. Mariano, and A.D. Taylor (2017). Colorful polymer solar cells employing an energy transfer dye molecule, Nano Energy, 38, 36-42.
T. Goh, D. Huang, T. Goh, J. Kong, A.D. Taylor, Z. Xu, S. Zhou, and Y. Zheng (2017). Perovskite solar cells with a DMSO-treated PEDOT:PSS hole transport layer exhibit higher photovoltaic performance and enhanced durability, Nanoscale, 9, 4236-4243.
G. Doubek, R.C. Sekol, J. Li, E. Moy, S. Nejati, W-H. Ryu, C. Reid, M. Carmo, M. Linardi, E. Kinser, Y. Liu, C.O. Osuji, J. Schroers, S. Mukherjee, and A.D. Taylor (2016), Guided Evolution of Bulk Metallic Glass Nanostructures: A Platform for Designing Three-Dimensional Bicontinuous Electrocatalytic Surfaces, Advanced Materials, accepted
F.S. Gittleson, D. Hwang, W-H Ryu, S. Hashmi, J. Hwang, T. Goh, and A.D. Taylor (2015), Ultrathin Nanotube/Nanowire Electrodes by Spin-Spray Layer-by-Layer Assembly: A Concept for Transparent Energy Storage, ACS Nano, 9 (10), 10005-10017.
F.S. Gittleson, K.P.C. Yao, D.G. Kwabi, S.Y. Sayed, W-H. Ryu, Y. Shao-Horn, and A.D. Taylor (2015), Raman Spectroscopy in Lithium-Oxygen Battery Systems, ChemElectroChem (Invited Mini Review for Special Issue; Very Important Paper), 2 (10), 1426-1426.
W.H. Ryu, F.S. Gittleson, M. Schwab, T. Goh, A.D. Taylor (2015), A Mesoporous Catalytic Membrane Architecture for Lithium-Oxygen Battery Systems, Nano Letters, 15, 434-441.
T. Goh, J-S. Huang, E. Bielinski, B. Thompson, S. Tomasulo, M. Lee, M. Sfeir, N. Hazari, and A.D. Taylor (2015), Co-evaporated Bi-squaraine Inverted Solar Cells: Enhancement Due to Energy Transfer and Open Circuit Voltage Control, ACS Photonics, 2 (1), 86-95.
X. Li, L.M. Guard, J. Jiang, K. Sakimoto, J.-S. Huang, J. Wu, J. Li, L. Yu, R. Pokhrel, G.W. Brudvig, S. Ismail-Beigi, N. Hazari and A.D. Taylor (2014), Controlled doping of carbon nanotubes with metallocenes for application in hybrid carbon nanotube/Si solar cells, Nano Letters, 14 (6), 3388–3394.
J-S. Huang, T. Goh, X. Li, M. Sfeir, E. Bielinski, S. Tomasulo, M. Lee, N. Hazari, and A.D. Taylor (2013), Polymer bulk heterojunction solar cells employing Föerster resonance energy transfer, Nature Photonics, 7, 479–485.
X. Li, Y. Jung, K. Sakimoto, T. Goh, M.A. Reed, and A.D. Taylor (2013), A Systematic Study for High Efficiency Single Walled Carbon Nanotube/Silicon Hybrid Solar Cells, Energy and Environmental Science, 6, 879-887.
R.C. Sekol, G. Kumar, M. Carmo, F.S. Gittleson, N. Hardesty-Dyck, S. Mukherjee, J. Schroers, and A.D. Taylor (2013), Bulk Metallic Glass Micro Fuel Cell, SMALL, 9 (12), 2081-2085.
M. Carmo, R.C. Sekol, S. Ding, G. Kumar, J. Schroers, and A.D. Taylor (2011), Bulk Metallic Glass Nanowires Architecture for Electrochemical Applications, ACS Nano, Vol. 5, No. 4, 2979-2983.
- Dr. Martin Luther King, Jr. Visiting Associate Professor, MIT, Cambridge, MA. (2014-2015)
- PECASE - Presidential Early Career Award for Scientists and Engineers (2011)
- Yale Greer Memorial Prize (2011)
- NSF CAREER Award (2010-2015)
I've been at Tandon since 2018, prior was at Yale (1st tenured AA in the history of the Yale School of Engineering and Applied Science), enjoy helping people win, prefer informal interactions but can adjust to the needs of the mentee.
My Science: Energy Devices, Nanostructured Materials, Organic Solar Cells
Research Briefs
Correlating wavelength dependence in LiMn2O4 cathode photo-accelerated fast charging with deformations in local structure
Electric vehicles are one of the best tools we currently have to combat the effects of climate change. There is one outstanding problem though: it takes a significant amount of time to charge a vehicle. Even fasting-charging units take much longer to “fill up” an EV than do gas pumps to fill a tank. This may seem like a mere annoyance, but for people pressed for time this is no small acceptance hurdle between internal combustion and EVs.
There have been numerous efforts at the material-level to improve charging rates through engineered electrode coatings and nanostructuring of active materials, which limit energy density in the battery pack. Additionally, the study of light interaction with energy storage materials has gained interest for use in photo-rechargeable batteries, and integrated solar energy storage systems, though there have been few studies specifically investigating light interaction with commercially-relevant battery materials. Facilitation of electron movement in a battery material is a key for lowering the resistance to flow of charge. Administration of light to induce light-matter interactions is a possibility to locally alter the electronic nature of the material. In particular, Spinel LiMn2O4 (LMO), a cathode material, was shown to dramatically increase the charging current when exposed to white light during a voltage hold.
Now, a team of researchers led by André D. Taylor, professor of Chemical and Biomolecular Engineering and member of the Sustainable Engineering Initiative, as well as graduated PhD student Jason Lipton and Christopher Johnson of the Argonne National Laboratory, have discovered how different wavelengths of light can change the current in LMO cathodes. The team showed that illuminating with red light results in a higher charging rate compared to both ultraviolet illumination of equal optical power and dark conditions.
The team analyzed the effect of red light in the context of the electronic structure and possible excitations, and showed that Mn d-d electronic transitions occurring under red light illumination are largely responsible for the increased charging rate. They further demonstrated through X-ray absorption spectroscopy methods that LMO Mn-Mn bond distances shorten after d-electron excitation. The shrinkage in the crystal volume beneficially contributes to delithiation kinetics by lowering the resistance to lithium-ion conduction.
The results provide a roadmap for rapid discovery of candidate materials for photo-accelerated fast charging, through the review of calculated density of states data. Besides LMO, there is a wealth of materials to investigate with strong potential for photo-electrochemically induced activity. Once photo-acceleration is optimized for a given materials system, it is possible to envision using a small amount of the source current from a fast-charging station to power thin, flexible LEDs built into the spiral wound 18650 cells in a battery pack, enhancing the fast-charging capabilities in next-generation EVs while minimizing impact to energy density.
Authors
- André Taylor
CO2 doping of organic interlayers for perovskite solar cells
The team reporting on this research was led by André D. Taylor, a professor of chemical and biomolecular engineering at NYU Tandon, and post-doctoral associate Jaemin Kong.
Perovskite solar cells have progressed in recent years with rapid increases in power conversion efficiency (from 3% in 2006 to 25.5% today), making them more competitive with silicon-based photovoltaic cells. However, a number of challenges remain before they can become a competitive commercial technology.
One of these challenges involves inherent limitations in the process of p-type doping of organic hole-transporting materials within the photovoltaic cells.
This process, wherein doping is achieved by the ingress and diffusion of oxygen into hole transport layers, is time intensive (several hours to a day), making commercial mass production of perovskite solar cells impractical. The Tandon team, however, discovered a method of vastly increasing the speed of this process through the use of carbon dioxide instead of oxygen.
In perovskite solar cells, doped organic semiconductors are normally required as charge-extraction interlayers situated between the photoactive perovskite layer and the electrodes. The conventional means of doping these interlayers involves the addition of lithium bis(trifluoromethane)sulfonimide (LiTFSI), a lithium salt, to spiro-OMeTAD, a π-conjugated organic semiconductor widely used for a hole-transporting material in perovskite solar cells, and the doping process is then initiated by exposing spiro-OMeTAD:LiTFSI blend films to air and light. Besides being time consuming, this method largely depends on ambient conditions. By contrast, Taylor and his team reported a fast and reproducible doping method that involves bubbling a spiro-OMeTAD:LiTFSI solution with carbon dioxide (CO2) under ultraviolet light.
They found that the CO2 bubbling process rapidly enhanced electrical conductivity of the interlayer by 100 times compared to that of a pristine blend film, which is also approximately 10 times higher than that obtained from an oxygen bubbling process. The CO2 treated film also resulted in stable, high-efficiency perovskite solar cells without any post-treatments.
The lead author Jaemin Kong explained that “Employing the pre-doped spiro-OMeTAD to perovskite solar cells shortens the device fabrication and processing time. Further, it makes cells much more stable as most detrimental lithium ions in spiro-OMeTAD:LiTFSI solution were stabilized to lithium carbonate, created while the doping of spiro-OMeTAD happened during CO2 bubbling process. The lithium carbonates end up being filtered out when we spincast the pre-doped solution onto the perovskite layer. Thus, we could obtain fairly pure doped organic materials for efficient hole transporting layers.”
Moreover, the team found that the CO2 doping method can be used for p-type doping of other π-conjugated polymers, such as PTAA, MEH-PPV, P3HT, and PBDB-T. Taylor said the team is looking to push the boundary beyond typical organic semiconductors used for solar cells.
"We believe that wide applicability of CO2 doping to various π-conjugated organic molecules stimulates research ranging from organic solar cells to OLEDs and OFETs even to thermoelectric devices that all require controlled doping of organic semiconductors,” and added that “Since this process consumes a quite large amount of CO2 gas during the process, it can be also considered for CO2 capture and sequestration study in the future. We are hoping that the CO2 doping technique could be a stepping stone for overcoming existing challenges in organic electronics and beyond.”
Authors
- André Taylor
Scalable, Highly Conductive, and Micropatternable MXene Films for Enhanced Electromagnetic Interference Shielding
Jason Lipton, a Ph.D. candidate under the guidance of Taylor, was lead author. Elisa Riedo (chemical and biomolecular engineering) and researchers from Drexel University and the Brookhaven National Laboratory also participated.
The proliferation and miniaturization of electronics in devices, wearables medical implants and other applications has made technologies for blocking electromagnetic interference (EMI) especially important, while making their implementation more challenging. While EMI can cause disruptions in communication in critical applications, resulting in potentially disastrous consequences, traditional EMI shields require large thicknesses to be effective, hampering design flexibility.
One solution resides in MXenes, a family of 2D transition metal carbides, nitrides, and carbonitrides with potential for blocking EMI demonstrate high conductivity and excellent EMI shielding properties. The key to the commercialization of these materials is industry-scale manufacturing.
A multi-institution research team led by Andre ́ D. Taylor, professor of chemical and biomolecular engineering at the NYU Tandon School of Engineering demonstrated a novel approach to MXene fabrication that could lead to methods for at-scale production of MXene freestanding films: drop-casting onto pre-patterned hydrophobic substrates. Their method led to a 38% enhancement of EMI shielding efficiency over conventional methods. The work suggests that micropatterned MXene films, prepared using a method that is scalable and allows for high throughput, can be readily used in EMI shielding, energy storage, and optoelectronics applications.
The team cast aqueous dispersions of MXene nanosheets (with the formula Ti3C2Tx) on hydrophobic polystyrene substrates and dried them. After drying, the resulting free-standing films could be easily peeled off, a method demonstrating a variety of advantages over the conventional vacuum-assisted filtration method with regards to time efficiency, operation simplicity, and surface smoothness.
The drop-casting method allows for modulation of micrometer-scale 3D patterns on the film surface by utilizing pre-patterned substrates (such as a vinyl record, retroreflective packaging, and retroreflective tape).
The research, “Scalable, Highly Conductive, and Micropatternable MXene Films for Enhanced Electromagnetic Interference Shielding,” is published in the first-anniversary issue of the Cell Press publication Matter.
Authors
- André Taylor,
- Elisa Riedo
Perovskite Solar Cells with Enhanced Fill Factors Using Polymer-Capped Solvent Annealing
Perovskite solar cells have seen massive improvements over the last few years. But despite big increases in power conversion efficiency, fill factors – one of the important characteristics in need of optimization – have still hovered around 80 percent, limiting the capacity for solar energy.
Thanks to a team led by Associate Professor André D. Taylor, that fill factor has been pushed up to 85 percent. Using a polymer-capped solvent-annealing process, they enhanced open-circuit voltage without sacrificing short-circuit current, creating better perovskite cells with improved output and a longer lifespan than current models.
The research team included NYU Tandon Postdoctoral Research Associates Jaemin Kong and Jason A. Röhr, along with colleagues from Yale University, Brown University, Brookhaven National Laboratory and the Korea Research Institute of Chemical Technology, and received funding from several groups including the National Science Foundation and the Office for Naval Research.
They found that during the solvent-annealing, the perovskite surface flattens and the perovskite grains agglomerate into micrometer-sized clusters having enlarged α-phase crystallites, while the δ-phase simultaneously disappears. The optimized structure enhances efficiency from 18.2 percent to 19.8 percent reliably, creating more stable and better solar cells.
Authors
- André Taylor