NYU Tandon researchers develop self-assembling, self-illuminating therapeutic proteins
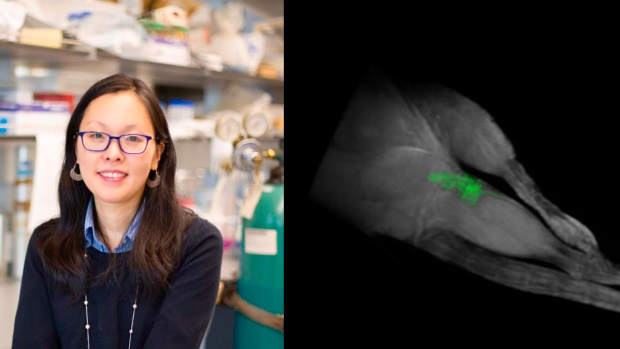
Researchers led by Jin Kim Montclare (left) have developed self-illuminating proteins used for delivering therapeutics to discreet areas of the body, as seen in a mouse's hindlegs (right).
When it comes to delivering drugs to the body, a major challenge is ensuring that they remain in the area they’re treating and continuing to deliver their payload accurately. While major strides have been made in delivering drugs, monitoring them is a challenge that often requires invasive procedures like biopsies.
Researchers at NYU Tandon led by Jin Kim Montclare, Professor of Chemical and Biomolecular Engineering, have developed proteins that can assemble themselves into fibers to be used as therapeutic agents for the potential treatments of multiple diseases. These biomaterials can encapsulate and deliver therapeutics for a host of diseases. But while Montclare’s lab has long worked on producing these materials, there was once a challenge that was hard to overcome — how to make sure that these proteins continued to deliver their therapeutics at the correct location in the body for the necessary amount of time.
In a recent study published by the journal American Chemical Society Applied Nanomaterials, her lab was able to create bio materials that were fluorinated. Thanks to this fluorination, they can be monitored by simple FMRI scans, allowing medical professionals to ensure that the drugs remain at the treatment areas through non-invasive imaging technology..
The material is made up of natural proteins, but the research team introduced the non-natural amino acid, trifluoroleucine. Because fluorine is rare in the body, it allows the biomaterials to light up like a holiday display when the body is put into an 19FMRI scan.
“As a theranostic agent, it can not only deliver a therapeutic for cancer or joint disease, for example, but we can now see that it’s still in place in the body and releasing the medication where it is supposed to,” Montclare says. “It removes the need for invasive surgeries or biopsies in order to see what’s going on.”
Montclare’s lab performs groundbreaking research in engineering proteins to mimic nature and, in some cases, work better than nature. She works to customize artificial proteins with the aim of targeting human disorders, drug delivery and tissue regeneration as well as create nanomaterials for electronics. Through the use of chemistry and genetic engineering, she has made contributions to diseases ranging from COVID-19 to osteoarthritis to many more.
This breakthrough uses the same amino acids and proteins that characterize much of Montclare’s research. Because they are made of organic materials, when these biomaterials have completed their job and delivered therapeutics, the body can break them down without any kind of adverse effects. This separates it from other treatments that use non-organic materials that could cause a severe immune response or other reactions. In combination with the fluorination technique, these materials could provide a treatment for localized diseases that can be far less invasive than current treatments and is far easier and less disruptive to monitor.
Montclare worked closely with NYU School of Medicine faculty on this study, including co-corresponding author Youssef Z. Wadghiri in the department of Radiology, as well as Richard Bonneau at the Flatiron Institute.
Montclare’s team showed their research in mouse models, but she is already looking to experiment on mice with specific disorders to prove the protein’s capabilities to treat diseases.
The self-assembling proteins that Montclare’s team used are only a subset of what she and her lab are working on. In another paper published in Biomacromolecules, her lab was able to use computational design to create proteins that could form hydrogels, thanks to a program written by her Ph.D. student Dustin Britton.
These hydrogels have different transition temperatures — the temperature that the gels can remain gelled without dissolving or becoming unstable. Previously, the upper limit of gelation was around 17 degrees Celsius. For biomedical applications, this was suboptimal, as it would melt as it approached human body temperature. Through the use of his computationally designed proteins, Britton was able to shift this limit up to 33.6 degrees Celcius.
Because of this new stability, the proteins that Britton and Montclare designed could be used for topical treatments, including healing wounds. And in addition to the increased heat tolerance, the new protein can gel much faster than previous versions, making it far more efficient and more useful for medical applications.
While shifting the temperature, Britton was also able to design a protein that is also fluorescent, meaning that it has the same potential for visualization as the fluorinated proteins in their other study. That allows doctors to monitor its presence in wounds and to ensure it’s delivering its therapeutic payload. And the gel has the same benefits of the lab’s proteins meant for internal use, in that it will be able to degrade and dissipate in the body with few-to-no ill effects.
Britton’s computer model is doing more than designing this specific protein. According to Monclare, the field of protein engineered biomaterials has long been dominated by trial-and-error — testing hypothetical designs hoping to see if they’ll be stable. But Britton’s model was able to create consistently successful gels, generating sequences with an extremely high success rate and creating new proteins with new properties for potential therapeutic uses.
“For biomaterial manufacturing, this will absolutely accelerate what we’re able to make,” says Montclare. “The way it’s traditionally done, you make rational changes and see if it works, and 90 percent of the time, it doesn't. With this new model, all of them work, and we can then pick from the best of the ones that work. It will revolutionize the way we make biomaterials.”
In Monclare’s lab, this has changed the way they’ll create new proteins and materials going forward — there's no going back to the rational iteration practice that had such a high failure rate. And it will surely accelerate the production of revolutionary biomaterials that will soon be healing some of the most serious medical conditions worldwide.