NYU Researchers Coax Colloidal Spheres To Self-Assemble Into Complex Photonic Crystals
Breakthrough Raises Hope for Efficient Alternative to Today’s Computer Chips
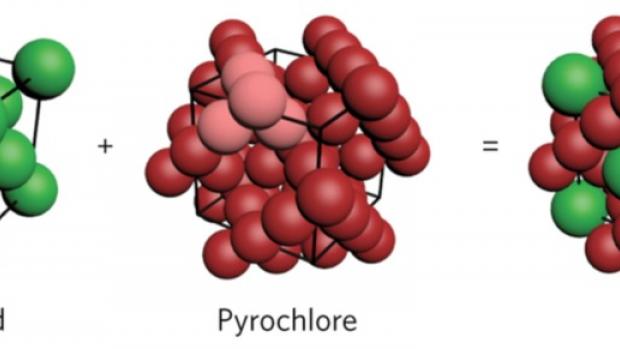
Colloidal particles, used in a range of technical applications including foods, inks, paints, and cosmetics, can self-assemble into a remarkable variety of densely-packed crystalline structures. For decades, though, researchers have been trying to coax colloidal spheres to arranging themselves into much more sparsely populated lattices in order to unleash potentially valuable optical properties. These structures, called photonic crystals, could increase the efficiency of lasers, further miniaturize optical components, and vastly increase engineers’ ability to control the flow of light.
A team of engineers and scientists from the NYU Tandon School of Engineering Department of Chemical and Biomolecular Engineering, the NYU Center for Soft Matter Research, and Sungkyunkwan University School of Chemical Engineering in the Republic of Korea report they have found a pathway toward the self-assembly of these elusive photonic crystal structures never assembled before on the sub-micrometer scale (one micrometer is about 100 times smaller than the diameter of a strand of human hair).
The research, which appears in the journal Nature Materials, introduces a new design principle based on preassembled components of the desired superstructure, much as a prefabricated house begins as a collection of pre-built sections. The researchers report they were able to assemble the colloidal spheres into diamond and pyrochlore crystal structures — a particularly difficult challenge because so much space is left unoccupied.
The team, comprising Etienne Ducrot, a post-doctoral researcher at the NYU Center for Soft Matter Research; Mingxin He, a doctoral student in chemical and biomolecular engineering at NYU Tandon; Gi-Ra Yi of Sungkyunkwan University; and David J. Pine, chair of the Department of Chemical and Biomolecular Engineering at NYU Tandon School of Engineering and a NYU professor of physics in the NYU College of Arts and Science, took inspiration from a metal alloy of magnesium and copper that occurs naturally in diamond and pyrochlore structures as sub-lattices. They saw that these complex structures could be decomposed into single spheres and tetrahedral clusters (four spheres permanently bound). To realize this in the lab, they prepared sub-micron plastic colloidal clusters and spheres, and employed DNA segments bound to their surface to direct the self-assembly into the desired superstructure.
“We are able to build those complex structures because we are not starting with single spheres as building blocks, but with pre-assembled parts already ‘glued’ together,” Ducrot said. “We fill the structural voids of the diamond lattice with an interpenetrated structure, the pyrochlore, that happens to be as valuable as the diamond lattice for future photonic applications.”
Ducrot said open colloidal crystals, such as those with diamond and pyrochlore configurations, are desirable because, when composed of the right material, they may possess photonic band gaps — ranges of light frequency that cannot propagate through the structure — meaning that they could be for light what semiconductors are for electrons.
“This story has been a long time in the making as those material properties have been predicted 26 years ago but until now, there was no practical pathway to build them,” he said. “To achieve a band gap in the visible part of the electromagnetic spectrum, the particles need to be on the order of 150 nanometers, which is in the colloidal range. In such a material, light should travel with no dissipation along a defect, making possible the construction of chips based on light.”
Pine said that self-assembly technology is critical to making production of these crystals economically feasible because creating bulk quantities of crystals with lithography techniques at the correct scale would be extremely costly and very challenging.
“Self-assembly is therefore a very appealing way to inexpensively create crystals with a photonic band gap in bulk quantities,” Pine said.
This research was funded by the U.S. Army Research Office under a Multidisciplinary University Research Initiative (MURI) grant.
Note: Images available at http://dam.engineering.nyu.edu/?c=1918&k=8596da3f0e
About the New York University Tandon School of Engineering
The NYU Tandon School of Engineering dates to 1854, the founding date for both the New York University School of Civil Engineering and Architecture and the Brooklyn Collegiate and Polytechnic Institute (widely known as Brooklyn Poly). A January 2014 merger created a comprehensive school of education and research in engineering and applied sciences, rooted in a tradition of invention and entrepreneurship and dedicated to furthering technology in service to society. In addition to its main location in Brooklyn, NYU Tandon collaborates with other schools within NYU, the country’s largest private research university, and is closely connected to engineering programs at NYU Abu Dhabi and NYU Shanghai. It operates Future Labs focused on start-up businesses in downtown Manhattan and Brooklyn and an award-winning online graduate program.