Dharik Mallapragada
-
Assistant Professor
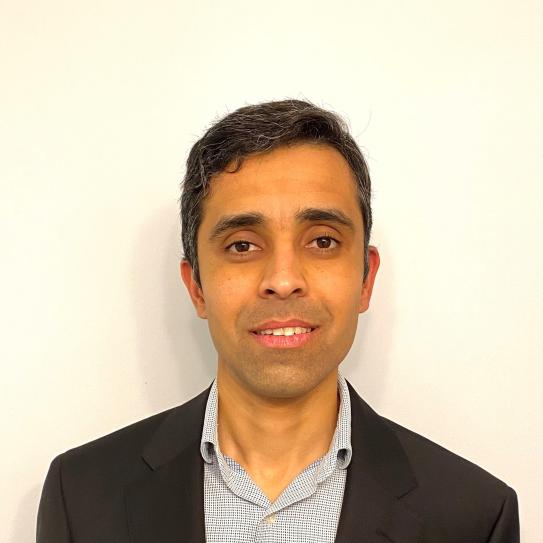
Energy system decarbonization requires widespread adoption of variable renewable energy-based electricity supply and electrification of many end-uses. Both these strategies will increase the spatial and temporal variability in energy supply and demand that complicates system operations and necessitates technological and market innovations to ensure reliable and cost-effective supply of low-carbon electricity. At the same time, for end-uses where electrification is currently impractical, there is a need to identify flexible and scalable processes for enabling electricity use or alternative emissions reduction strategies that can complement grid decarbonization efforts.
The Sustainable energy Transitions (SET) group is interested in developing mathematical modeling approaches to analyze low-carbon technologies and their energy system integration under different policy and geographical contexts. The group’s research aims to create the knowledge and analytical tools necessary to support accelerated energy transitions in developed economies like the U.S. as well as emerging market and developing economy countries in the global south that are central to global climate mitigation efforts. We develop mathematical models for design and operations of processes and integrated energy systems. We use a wide range of computational techniques in our work including first-principle and data-driven modeling, formulating and solving mixed integer linear/nonlinear optimization models and data analytics for variability and uncertainty characterization.
Education
Indian Institute of Technology Madras, 2008
B.Tech, Chemical Engineering
Purdue University, 2013
M.S, Chemical Engineering
PhD, Chemical Engineering
Recent Publications
- Khorramfar, R., Mallapragada, D., and Amin, S. (2024). Electric-gas infrastructure planning for deep decarbonization of energy systems. Applied Energy 354, 122176. 10.1016/j.apenergy.2023.122176.
- Schittekatte, T., Mallapragada, D., Joskow, P.L., and Schmalensee, R. (2024). Electricity Retail Rate Design in a Decarbonizing Economy: An Analysis of Time-of-use and Critical Peak Pricing. The Energy Journal 45, 25–56. 10.5547/01956574.45.3.tsch.
- Schittekatte, T., Mallapragada, D., Joskow, P.L., and Schmalensee, R. (2023). Reforming retail electricity rates to facilitate economy-wide decarbonization. Joule 7, 831–836. 10.1016/j.joule.2023.03.012.
- Riedmayer, R., Paren, B.A., Schofield, L., Shao-Horn, Y., and Mallapragada, D. (2023). Proton Exchange Membrane Electrolysis Performance Targets for Achieving 2050 Expansion Goals Constrained by Iridium Supply. Energy Fuels 37, 8614–8623. 10.1021/acs.energyfuels.3c01473.
- Zang, G., Graham, E.J., and Mallapragada, D. (2023). H2 production through natural gas reforming and carbon capture: A techno-economic and life cycle analysis comparison. International Journal of Hydrogen Energy. 10.1016/j.ijhydene.2023.09.230.
- Barbar, M., Mallapragada, D.S., and Stoner, R.J. (2023). Impact of demand growth on decarbonizing India’s electricity sector and the role for energy storage. Energy and Climate Change 4, 100098. 10.1016/j.egycc.2023.100098.
- Sheha, M., Graham, E.J., Gençer, E., Mallapragada, D., Herzog, H., Cross, P., Custer, J., Goff, A., and Cormier, I. (2024). Techno-economic analysis of a combined power plant CO2 capture and direct air capture concept for flexible power plant operation. Computers & Chemical Engineering 180, 108472. 10.1016/j.compchemeng.2023.108472.
- Mallapragada, D.S., Dvorkin, Y., Modestino, M.A., Esposito, D.V., Smith, W.A., Hodge, B.-M., Harold, M.P., Donnelly, V.M., Nuz, A., Bloomquist, C., et al. (2023). Decarbonization of the chemical industry through electrification: Barriers and opportunities. Joule 7, 23–41. 10.1016/j.joule.2022.12.008.
In the News
- Unlocking hydrogen’s potential for renewable energy storage, transport (Lehigh)
- This key chemical is super dirty to make. Can an electric furnace help? (Canary Media)
- To decarbonize the chemical industry, electrify it (MIT News)
- How the hydrogen revolution can help save the planet — and how it can’t (Nature)
- As energy costs soar, homeowners are seeking ways to stay warm without breaking the bank (Boston Globe)
- In the transition to renewables, energy storage is a hot topic (MarketPlace)
- Meet the research scientists behind MITEI’s Electric Power Systems Center (MIT News)